1.3
Characteristics and general aspects of small satellites
The term “small satellite” has a universal definition, but can be expressed in different ways. These can be physical parameters (weight, dimensions) or unique design and operating parameters.
As previously mentioned, the concept of small satellites is connected with issues related to the requirements for the use of radio spectrum, and orbit, along with obligations arising from the implementation of international agreements on the use of space.
Given these facts, we will continue to use our needs as the category of small satellites used in the administrative processes at OOSA (registration at COPUOS) and ITU (notifications, management of radio spectrum and orbits according to the Radio Regulation).
- minisatellites - less than 1000 kg,
- microsatellite – less than 100 kg,
- nanosatellite – less 10 kg,
- picosatellite – less 1 kg,
- femtosatellite – less 0,1 kg.
For example, another detailed breakdown is used by NASA for the CubeSat standard category (https://www.nasa.gov/content/what-are-smallsats-and-cubesats):
- minisatellite - 100-180 kg,
- microsatellite - 10-100 kg,
- nanosatellite - 1-10 kg,
- picosatellite – 0,01-1 kg,
- femtosatellite – 0,001- 0,01 kg.
The arrangement of CubeSat of the small satellites used a modular compose the basic unit called 1U (pico-satellite 1 kg) to the configuration shown in Figure 8.
+
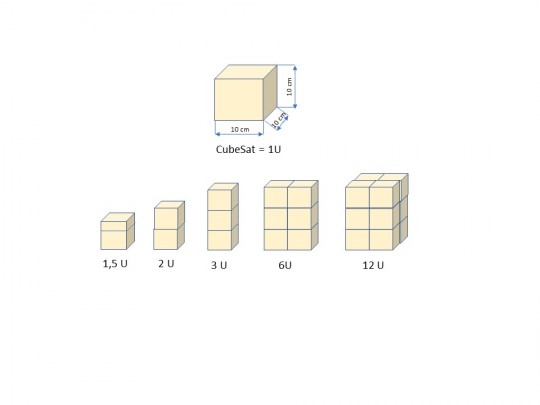
Fig. 8. Modular design of CubeSat satellites
A group of characteristic features is used to define the concept of a small satellite:
- short development time,
- small teams development teams,
- small development and testing infrastructure,
- acceptable investment costs for development, construction, launching, deployment and operation,
- use of low LEO orbit up to 1500 km on the Earth,
- short operating time - from several months to 3 years.
A small satellite can also be described using this summary terms for our needs:
Definition
A small satellite is an artificial body that uses space on low orbits, which is small in size and weight, is made quickly and inexpensively, and has short lifetime.
Now, in the basic features, we will note the individual characteristics of the small satellite above. They will be discussed in more detail in the following separate chapters.
Add a) Short development time
The development time of a small satellite can be shortened considerably by using standard components (so-called “off-the-shelf”) on the market (e.g. batteries, antenna material, radio equipment), by purchasing ready-made and tested small satellite kits, and by producing custom components, for example on the 3D printer. This benefits the construction of the satellite and its payload various technological or scientific experiments. Further development shortening can be achieved by limiting the time and number of pre-start tests (testbed).
Add b) Development teams with a small number of people
The availability of the shelf components, modular design, small size, and low satellite weight (especially nano, pico, and femto satellite´s category) allow the realization of projects created by teams of a small number of specialists. During the entire process, from planning to operation, these teams can flexibly change and simultaneously participate in more projects. The school teams welcome this flexibility which allows them to build small satellites and carry out experiments. There is also the possibility of setting up start-ups, small and medium-sized enterprises for the development, production, and sale of components for small satellite kits.
Add c) Small range of development and test infrastructure
The development time and the number of prototype tests is reduced due to the use of conventional components whose functionality has been tested. The test does not need to be highly specialized with expensive measuring instruments and test equipment, including rental of specialized certified laboratories. Thus, only the pre-launch tests (testbed) according to the provider's requirements for launching a small satellite into orbit remain necessary.
Add d) Acceptable investment costs for development, construction, launch, and operation
Considering the previously mentioned factors and thanks to new possibilities, the involvement of the private sector to launch small satellites and dock with the ISS technical platform for the release of small satellites have created favorable conditions to reduce the necessary financial resources and the realization of small school projects. However, it is clear that regardless of the type of small satellite, the cost will be in the order of tens of thousands of US dollars.
A favorable fact from the financing point of view is that due to the possibility of launching more small satellites at one launch of the launcher, the cost of launching is shared among multiple entities. Additionally, the option of adding to the payload when sending large satellites will reduce the financial burden. Especially for school projects and for educational purposes, discounts are also provided.
Also, a small satellite does not need an expensive ground station or secondary or multiple backup stations to operate. Practically amateur radio stations supplemented by suitable preamplifiers, together with rotating directional antennas, can be used. All this is readily available on the Czech market at the price of 200 000 CZK.
The term "acceptable amount" may seem like a rather vague, however, the financing is spread over time. While it is a matter of months in these missions, during this time, for example, there may be changes in financial support. As a result, the launcher does not launch at the scheduled time, fails to deploy a small satellite in the planned orbit, doesn't expand the antennas or photovoltaic panels, radio communication equipment breaks down, etc.
Add e) Utilizing LEO orbit up to 1500 km on Earth
The small satellites that are the subject of this material operate in low orbits (LEOs) and are mostly of the polar orbit type (fly over the Earth's poles).
The use of low orbits allows global coverage of radio communication between 98 ˚ north and 98 ˚ south latitude. Thus, a small satellite does not require to carry powerful radio communications, which are cheaper. Transmissions with less power reduce demands on rechargeable batteries and, therefore, also on the performance of photovoltaic cells. It is also possible to use less expensive antenna systems with less weight.
All of these factors lead to more space for more experiments. Due to the short propagation path, the radio signal has a low latency. Options must be added which allow the deployment of small satellites from the ISS.
However, LEO orbits allow for a short communication time with a small satellite for about 10 minutes and an orbital time depending on the altitude of about 80 to 130 minutes. This communication time at the ground station requires the use of rotating directional antennas and the elimination of the Doppler effect. It also causes the transmit and receive frequencies to shift during the overflight of small satellites. The communication time requires that, when tracking the satellite, the changes in the rotating antenna systems are synchronized with the radio transmitter and receiver frequencies.
Add f) Short operation time
Small satellite missions generally have a short lifetime in orbit. This lifespan is planned or unplanned. For example, for school projects, the planned operating time is usually up to one year. Maximum lifetime of a mission is expected within three years.
Unplanned termination of a mission after a successful launch may be due to non-location or premature loss of orbit, the use of common components such as conventional Li-Ion rechargeable mobile phone batteries, or failure deployment photovoltaic panels or antennas, flight software error, etc. These factors can also significantly shorten the mission. However, there may be many other causes. Some will be discussed in more detail in the following chapters.
For further explanation, it will be useful to give some theoretical knowledge related generally to the movement of artificial objects in space. Furthermore, with parameters that are the subject for planning and practical implementation of small satellite missions, and one will be discussed in more detail in the next chapters.
Depending on the altitude, the following orbital division is used:
- LEO (Low Earth Orbit): 160 km – 2000 km (see Fig. 7), this is used by small satellites (CubeSat, Orbcomm, meteorological satellites, Earth´s monitoring satellites, etc.) and it is the subject of this material,
- MEO (Medium Earth Orbit): 2000 km – 35 786 km, it is used by telecommunications (mobile networks Inmarsat, Iridium, Global Star), navigation (GPS, Galileo, Glonass, Beidou), sensor monitoring, etc.,
- HEO (High Earth Orbit): greater than 35 786 km geostationary but a much lower perigee, rarely used,
- HEO (Highly Elliptical Orbit): the farthest point on the track satellites (apogee) is greater than 35 786 km, extra high orbital elliptical eccentricity, used mainly Russian telecommunication services of satellites Molnyia,
- GEO (Geostationary orbit) – greater than 35 786 km, used for TV and R broadcasting, and fixed services (Internet, data networks, etc.),
- Lunar orbit: more than 384 401 km (probes and missions to the Moon and planets).
Three laws formulated by Johann Kepler between 1609 and 1619 generally govern the shape of the orbits and tracks of the satellites in space. These describe the patterns of planetary motion around the Sun and are applied to the motion of satellites around the Earth.
From the First Law (the “Orbit Law”) states that satellites move around the Earth on elliptical orbits.
From the second law (the "law of surface") states that the circumscribed areas are identical when the satellite moves around the Earth at the same time.
The third law (the "orbital time law") states that the square of the orbital time (T) is equal to the square of the length of the major axis of its orbit. For clarification of this relationship is used the standard gravitational parameter μ including the effect of the Earth.
The relation: µ = 4.π2.a3/T2 a – length of the semi-major axis of the ellipse (1)
Follows this formula is then possible to calculate, for example, the orbital time (orbital period) T0 of a small satellite:
(2)
where µ is the product of the gravitational constant G and the mass M of the Earth and is equal to 3.986x1014 m3s-2
The following Kepler´s elements are derived and used from the above laws:
- eccentricity - deviation (offset) from the circular orbit
- semi-major axis – half of the major ellipse axis
- inclination – the difference between the satellite´s orbital plane and the equator
- argument of perigee – number of degrees around the 360˚ orbit from a reference point (the ascending node) to the point where the satellite is at the closest to the Earth (perigee)
- argument of the most distant point of the satellite to the Earth (apogee),
- rectascenze the ascending path node (RAAN – Right ascension of the ascending node) – the angle measured eastward from the “First point of Aries” reference line to the first ascending node. An ascending node is the where the satellite crosses equator while moving from South to North.
This has two complementary elements used for prediction and tracking satellites, which are:
- epoch – calendar data (day, month, year)
- drag – a force against the movement of a satellite due to its movement in an environment that resists. This is particularly important for LEO orbits. LEO orbits reach the upper layers of the ionosphere and the magnetosphere (see Fig. 7). As a result, it causes the satellite to gradually decelerate and thereby move away from its orbit (where it would otherwise move infinity) towards the atmosphere. As a result of its extinction by burning in the upper atmosphere.
+
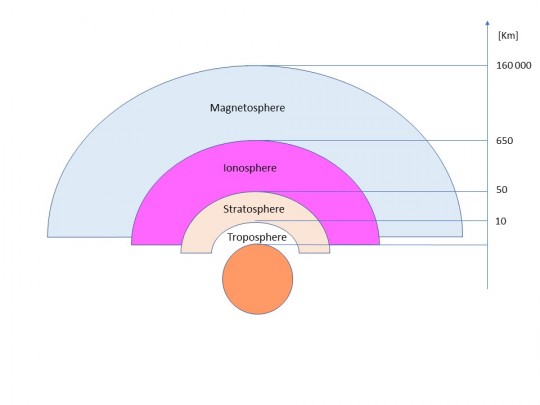
Fig. 9. Layers of the Earth's atmosphere
Satellite motion and relationships that are used in the discussion that follows are shown in Fig. 10.
+
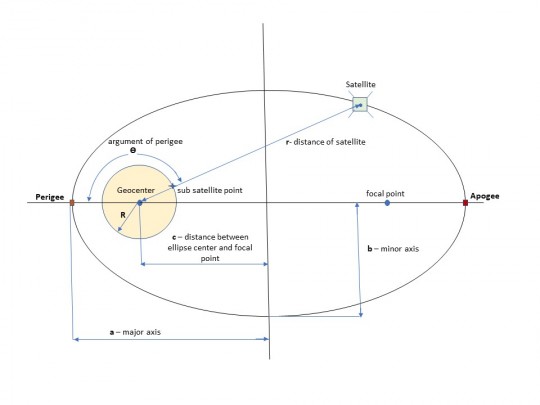
Fig. 10. The movement of a satellite around the Earth
The following relationships apply:
Eccentricity in orbit: E = √(1-(b/a))2 0 ≤E <1 (3)
For the distance of apogee ra and perigee rp from the Earth's geocentre:
ra = a(1+E) [km; km] (4)
rp = a(1-E) (5)
Orbital period To can be expressed previously mentioned formula (1).
For velocity in orbit vo, which is generally variable, the following formula applies:
vo= √(GM(2/r)-1/a) [m/s; m] – a length of semi-major axis (6),
where GM is the product of the gravitational constant G and the mass M of the Earth and is equal to 3.986x1014.
The expression of the motion of the satellite orbit are used by many other parameters of the connection are shown in Figs. 11 to 13. The track inclination parameter is shown in Figure 11 and can be 0˚ – 90˚ and 90˚ – 180˚.
+
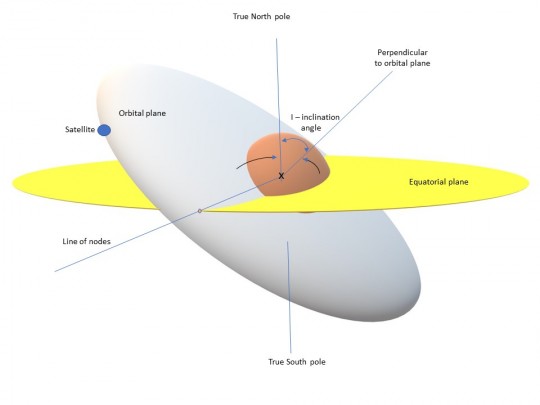
Fig. 11. An orbit inclination image
Another orbit parameter is the perigee argument shown in Figure 12.
+
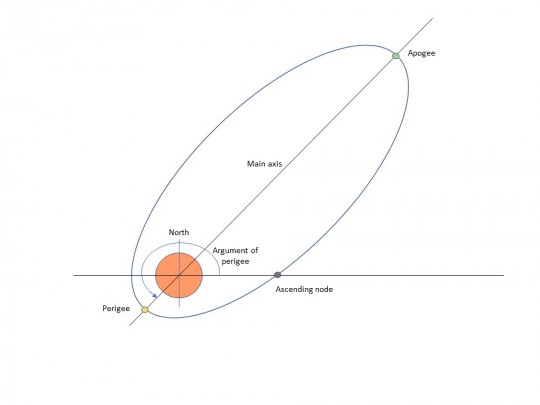
Fig. 12. A graphical representation of perigee argument
Examples of orbits for 0˚, 90˚ and 45˚ inclinations are shown in Figure 13.
+
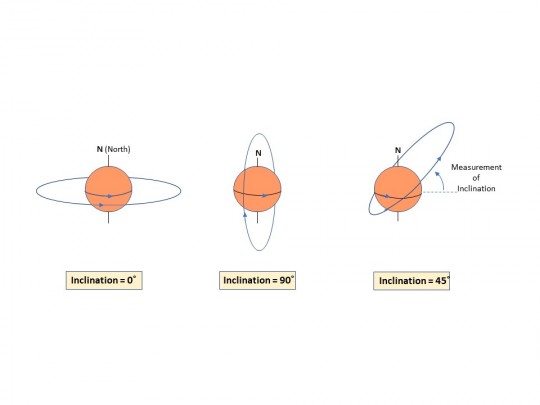
Fig. 13. Examples of orbit inclinations - 0˚ (equatorial orbit), 90˚ (polar orbit)
Small nano, pico, and femto satellites mostly use LEO orbits with tilt around 97° – 98˚. Inclinations higher than 90˚ consequently have the direction of motion of the satellite is in the track against the direction of rotation of the Earth. The earth rotates from West to East, counter-clockwise.
Another important parameter of a satellite is how much surface area of the Earth a signal covers or where and how long is it possible to communicate with the satellite.
To receive radio signals at the ground station, whose location is expressed in latitude and longitude, the satellite must be over the earth's horizon for a time. We distinguish an optically visible horizon from a radio horizon.
For communication with satellites, frequencies above 50 MHz, which propagate similarly to light on straight orbits, are used. Under normal propagation conditions the value of the radio horizon is approximately 15 % greater than the optically visible horizon.
Distance to the radio horizon LRH depending on antenna height (h) above the ground can be calculated by the formula:
Lrh= √(17. ha) (8)
At low elevation angles above the horizon, satellites in exceptional propagation conditions can reach greater distances than the above relation (8).
Radio coverage from the satellite on the Earth's surface is indicated by the term "footprint." Its representation is in Figure 14.
+
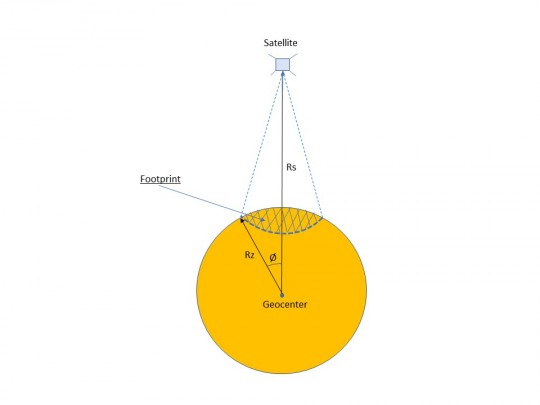
Fig. 14. Coverage of the Earth's surface with a radio signal of the satellite - footprint
The maximum number of possibilities for realization of radio communications from the ground station can be expressed as follows for the size of the footprint surface Sf and its radius Rf.
Maximum možnosti uskutečnění rádiové komunikace z pozemní stanice je možné vyjádřit následujícími vztahy pro velikost plochy Sf (km) a footprint diameter Rf.
S f= 2πRz2 x (1-cos (ɸ)) [km2] (9)
Rf=Rz x arccos (Rs /Rz) x 2 [km] (10)
The above characteristics and relationships should give a basic idea of what a small satellite is and how it moves in space. It should be remembered that these are basic theoretical relationships. They do not include other influences and anomalies that need to be covered under real conditions. However, we do not have to take them into account in order to introduce small satellites issues.
Of course, when planning a project of the mission itself, it is necessary to deal with small satellites and also to define additional parameters. Some of them will be described in successive chapters.